Often presented as a clean alternative to fossil fuels, biofuels may turn out to be a false solution to the climate and energy crises
On hearing the word biofuel, it is hard not to be hit by its two-headed-monster nature. Although the prefix bio (from the Greek root for “life”) suggests something in harmony with living processes, the word fuel has become associated with the members of a wicked family of substances responsible for some of the darkest crises humanity has ever faced. As it turns out, not only the term biofuel, but also its value as a safe alternative to fossil fuels, generates animated discussions. Fuels of biological origin, alongside and even more than other renewable energy resources, have become a central player in two of the most controversial issues of our time: the rising of inequality and the climate crisis.
In this non-technical, yet fully-referenced article, I will address:
what pushes scientists to look for alternative fuels;
how energy is stored in fuels;
what biofuels are;
how they are made and why they are less harmful than fossil fuels;
the science behind why they might not be a solution at all.

1. The quest for sustainable energy
The scientific community and society as a whole have been in search of an alternative to fossil fuels for several decades. Even though there is an ongoing debate about how much oil remains to be extracted¹, the link between the burning of fossil fuels and the rise of global average temperatures has become increasingly more evident². In a recent scientific assessment³, it was argued that…
…to save millions of lives and restore aerosol-perturbed rainfall patterns, while limiting global warming to 2 °C, a rapid phaseout of fossil-fuel-related emissions and major reductions of other anthropogenic sources are needed.
Such conclusions have led to a call to replace fossil fuels with emission-free alternatives. Such alternative fuels are required to satisfy at least two environmental criteria:
they should not cause the release of carbon dioxide (CO₂) in the atmosphere (zero net carbon),
they should come from renewable resources (which are able to replenish over human time scales).
Moreover, notable efforts have been made to find solutions that are:
available at affordable prices (compared to oil).
suitable for the transportation sector,
capable of supplying a significant portion of the global energy demand.
The last two criteria listed above have been questioned by activists, economists, politicians and scientists who claim that the only viable solutions to the climate crisis will require a complete restructuring of the current production system. In their view, public institutions should implement a reduction of the global energy consumption rate⁴, rather than maintaining a “green version” of the current one. However, even in this scenario, since fossil fuels currently account for 80% of global consumption⁵, any gradual reduction would still require a transition from fossil fuels to suitable alternatives.
Several sustainable ways of generating electrical power have been developed and are currently deployed⁶; they transfer energy from gravity (hydropower), wind, the sun (photovoltaic), the inside of the earth (geothermal), sea waves and tides. However, none of these represents a solution to the fuel problem, at least until most transportation systems, as well as industrial and agricultural machinery, are not powered directly by electricity.
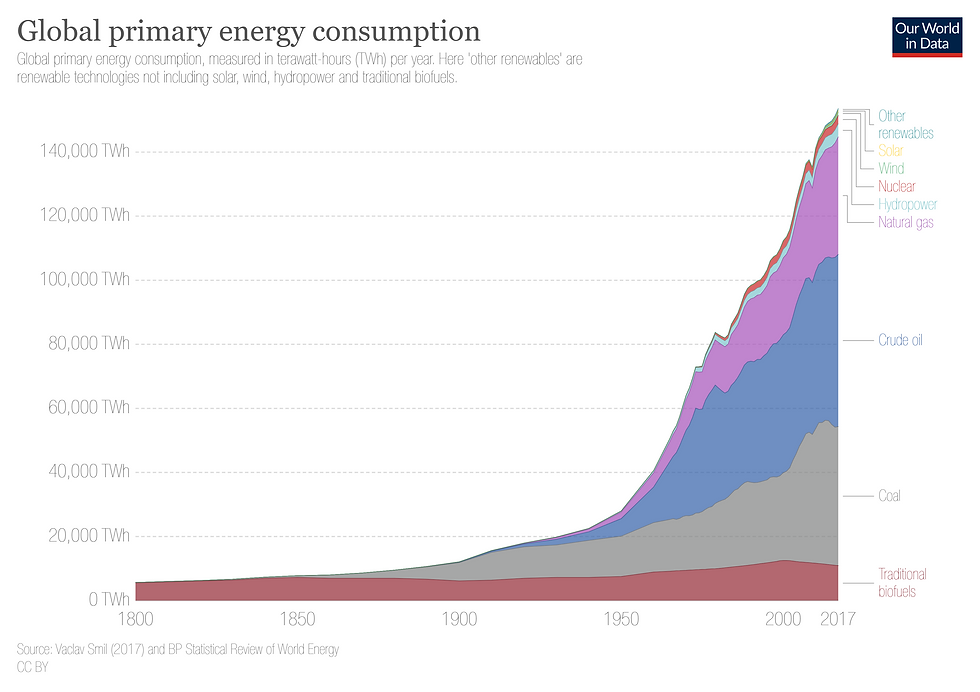
Figure: Global primary energy consumption. Source and interactive chart at OurWorldInData.
2. Photosynthesis: how sunlight becomes fuel
Given the undisputed (and disastrous) success of fossil fuels, it can be helpful to look at what makes them so unique. Where does the energy stored in oil, coal and natural gas really come from?
Actually, it comes from the sun⁷. Starting as early as 2 billions of years ago⁸ on a slightly younger planet earth, algae and land plants converted, as they continue to do now, solar energy into a chemically storable form, taking advantage of the properties of the element carbon. The process starts with photosynthesis, the transformation of water and carbon dioxide into sugar (glucose); here, sunlight energy is used to bind together (or “fix”) the atoms that make up the chemical structure of glucose. Part of the glucose thus produced is then processed in order to provide plants with energy (in the form of ATP, via respiration). However, a considerable part of the carbon, hydrogen and oxygen are recombined into storable carbon-rich (organic) compounds, such as lignin, amylase (starch) and cellulose (dietary fibre)⁹. We refer to these stores as biomass, plant tissues or organic matter.
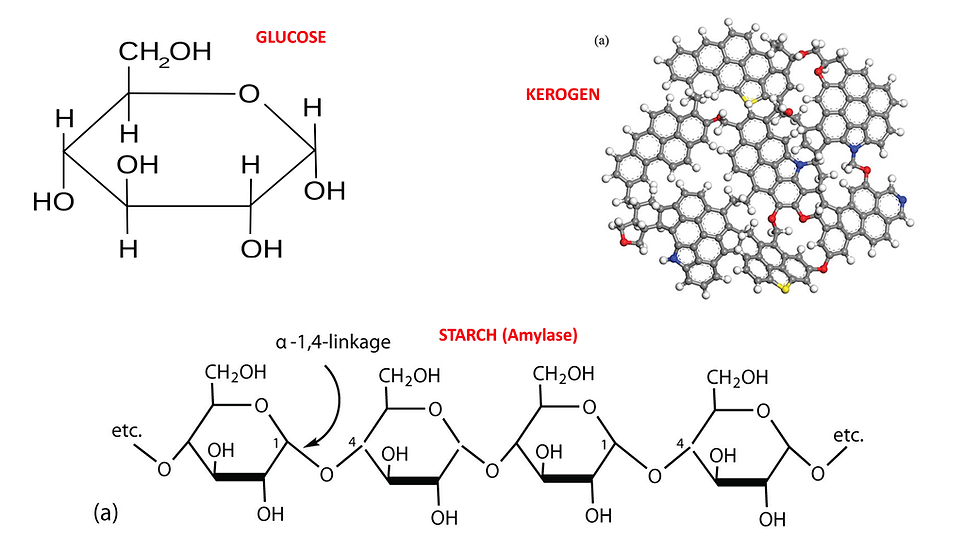
Figure: Three examples of organic compounds. Glucose is produced in photosynthesis; Starch is stored in plant tissues; Kerogen, a precursor of oil, is a concentrated store of energy with its many carbon bonds.
Nature has developed its own ways to cycle this biomass back into the ecosystem it belongs to:
The quickest method is aerobic decomposition¹⁰, which is carried out in oxygen-rich environments by the soil food web. When animals eventually die, their store of carbon-rich compounds is deposited on the surface of the soil, together with dead vegetation. Worms, insects, bacteria, fungi and other microorganisms take care of this organic matter, thus separating it into soluble plant nutrients, atmospheric gases and humus. Each of these bioproducts is re-used within the ecosystem to sustain one of its functions: soluble minerals and atmospheric carbon feed plants (enabling photosynthesis), and humus provides cosy living conditions for the soil food web’s members¹¹.
Where oxygen is not present, anaerobic decomposition¹² takes place instead. This is common in waterlogged soils, bogs, lakes and at the bottom of oceans. Acidogenic bacteria, fungi and other microbes thrive in these oxygen-deprived environments, where they process organic compounds to produce methane and carbon dioxide while re-cycling nitrogen and sulfur¹³ into the soil and the atmosphere;
However, if anaerobic decay occurs at high temperatures and under enormous physical pressure, something very different happens. Indeed, in peatlands or oceanic seabeds, layers of organic matter are compressed by the water above and undergo a biochemical transformation that can last up to 650 millions of years.⁷. This process, in which organic compounds are completely deprived of their oxygen atoms, leads to the formation of fossil fuels¹⁴. Where the decayed material comes mostly from terrestrial plants, as at the bottom of peatlands, coal and natural gas form; on the other hand, aquatic vegetation leads to the formation of a waxy material known as kerogen, which is found in oil shales and further into oil and natural gas¹⁵. Thus, carbon (and the energy stored in its bonds with hydrogen) exits the ecosystem and is stored in the form of mineral substances embedded in sedimentary rocks, at depths from 7 to 100 metres deep¹⁶.

3. Four generations of Biofuels
As humans, we convert the energy stored in plant tissue whenever we eat and digest vegetables; digestion (by gastrointestinal microorganisms) and respiration (in cells’ mitochondria) can transform organic matter into soluble and easy to transport ATP. However, if we are interested in utilising the energy converted by photosynthesis as fuel, we can either process plant tissue directly into biofuels, or burn fossil fuels.
Fossil fuels Although photosynthesis is the common underlying mechanism, fossil fuels are a concentrated storage form of former biomass, and thus have a high energy density. On the other hand, fossil fuel formation requires millions of years, and our current burning rate does not allow them to replenish fast enough¹⁵.
Biofuels There is obviously the possibility to use organic matter from plant and animal origin directly, by processing it in a way that releases the energy it stores in its chemical bonds. The refined product of this chemical transformation is called a biofuel¹⁶.
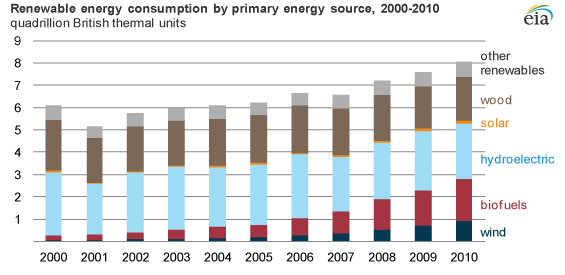
A biofuel is a solid, liquid or gaseous fuel derived from any biological carbon source including treated municipal and industrial wastes¹⁷.
Human beings have been utilizing bioenergy and biofuels for domestic purposes since pre-recorded history. Wood was burned for heat and light to cook food, illumine night, warm shelters, and treat clay artifacts.³²
Since Biofuels are produced directly from organic matter, they have oxygen levels from 10% to 45%, as opposed to oil that has none. Moreover, they have a higher content of hydrogen (or high H to C ratio) too. This makes the chemical processing and the properties of biofuels very different from those of fossil fuels.
Numerous studies and a vast amount of financial and human resources have been dedicated to the challenge of devising viable, efficient processes that can also be scaled up to compete with fossil fuels on the market¹⁸. We have thus been able to witness, within a few decades, the development of four generations of biofuels¹⁹:
The first, traditional source of biologically produced fuels are food crops such as wheat, sugarcane and soybeans. The sugar, starch or oil derived from these crops are usually converted into fuel via yeast fermentation²⁰;
The second generation of biofuels is constituted by non-food crops, such as grasses, woody crops and agricultural waste (stems, stalks, leaves). This material is usually referred to as lignocellulosic, as it contains lignin and cellulose. These can be processed in several ways, but are generally heated (thermochemical treatment) either in the presence or absence of oxygen²⁰;
Third-generation biofuels were introduced in the 1980s, and are derived from algae, bacteria and other microorganisms⁶⁹.
Finally, fourth-generation biofuels have recently drawn the attention of venture capitalists and green growth enthusiasts. These are produced from genetically modified algae that contain high quantities of fats (lipids). Although algal biofuel has attracted huge interest and investment in the last two decades, their production on a large scale has yet to be implemented, due mostly to economic viability issues²¹.
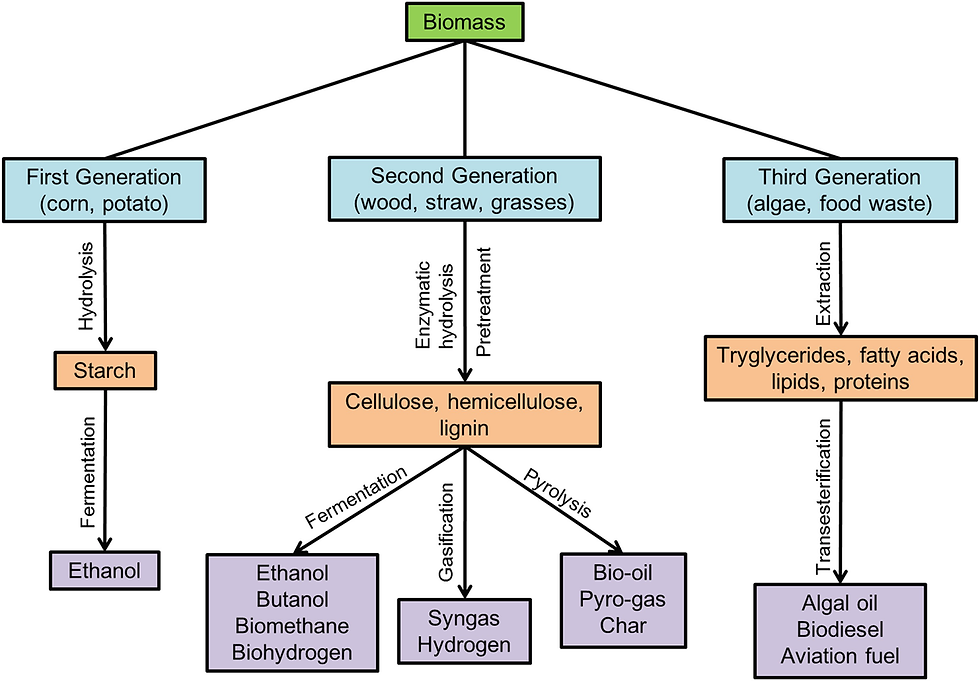
Figure: Different generations of biofuels¹⁹
3. How biofuels are made
Thermochemical processing of biomass
Perhaps the most common way of tapping into the energy stored within plant biomass is by heating it up or burning it (thermal conversion) or composting it (biochemical conversion). In both cases, carbon bonds are broken to release energy in the form of heat. Thermal conversions include the burning of wood that was the primary source of energy for millennia, as well as modern gasification of organic matter³⁰. The process underlying the production of gas from biomass is called pyrolisis (in essence similar to the production of charcoal) and can be performed to different degrees of completion, depending on the oxygen allowed in the reaction. Syngas, for instance, is derived from incomplete pyrolysis.
In 2002, 10–14% of energy production came from biomass conversion³¹. Currently, common sources of biomass are willow, poplar, Miscanthus and switchgrass (Panicum virgatum). The key to making biomass conversion efficient is to find suitable species, which have a high content of carbon- and energy-rich lignin, and do not require vast areas of land to be grown.
Biogas from anaerobic digestion
Biogas is a process that mimics anaerobic decay of organic matter as it occurs in naturally oxygen-poor conditions. This anoxic environment is recreated either in a landfill or in a digester (sometimes called bioreactor); here, food waste, animal manure in the form of liquid slurry or non-food crops such as maize silage are left in the company of millions of anaerobic bacteria³⁴. The process, which is similar in character to an unpleasantly flatulent human digestion, produces methane, carbon dioxide and carbon monoxide, which can be combusted as a gaseous fuel.
Biogas production has two obvious benefits: it recycles potentially hazardous waste (in particular animal slurry), and it puts to good use the methane and nitrous oxide that animal manures produce when they are let to decompose slowly³⁷.
However, sulfur compounds and ammonia gases are produced as a polluting byproduct³⁷.
Biodiesel from vegetable oil extraction
Biodiesel is a biofuel made from plant or animal-based fats and oils. Common plant sources are sunflower, coconut and the African oil palm. As the name suggests, it is a drop-in fuel that can be fed into a conventional diesel engine. In fact, the original prototype of a Diesel engine used peanut oil as a fuel³⁹.
Biodiesel is produced by mixing together alcohol (e.g., ethanol) to biologically sourced fats. If a catalyst (as potassium hydroxide) is added and heat is supplied, a chemical reaction (called transesterification) ensues⁴⁰. The products of the reaction are biodiesel (ethyl esters) and glycerol, which needs to be separated and removed to obtain pure fuel.
Biodiesel has been acclaimed for its energy content (or calorific value), which is only 9% lower than conventional petrol-derived diesel⁴¹, and its high lubricity, which reduces engine wear and makes it an even more versatile substance.
In addition to this, pure biodiesel (B100) has been shown to emit 75% less carbon dioxide than petrodiesel, and has a lower market cost⁴².

Figure: Biodiesel production
Bioethanol from fermentation
Bioethanol was introduced on a large scale in the 1980s in Brazil, where it has been a worthy competitor of fossil fuels ever since. It can be produced starting from starch or sugars from land plants or from algae. Sugar cane and corn are the primary sources⁴³.
Bioethanol production requires three steps: yeast fermentation (which is a similar process to the naturally occurring anaerobic decay) distillation and dehydration to achieve suitable purity. Sugars, as well as enzymes, are needed during the fermentation phase; the enzymes break cellulose and trigger the recombination of carbon and hydrogen atoms into short-chain alcohol molecules. In recent years considerable work has been done on improving the pretreatment of the biomass input to reduce enzyme requirements, in combination with genetically engineered plants⁴⁴. Due to its oxygen content and its simple structure, bioethanol burns with virtually no contaminant byproducts and at lower temperatures than petrol and biodiesels⁴⁵.
Algal biofuel
Algae are unicellular (micro-algae) or multicellular (macro-algae) primitive forms of plants, that are able to photosynthesize and thud converting sunlight, CO₂ and H₂O to lipids, proteins, carbohydrates and hydrogen. They are found in fresh and salty water and possess an enormous potential for conversion into usable fuels.
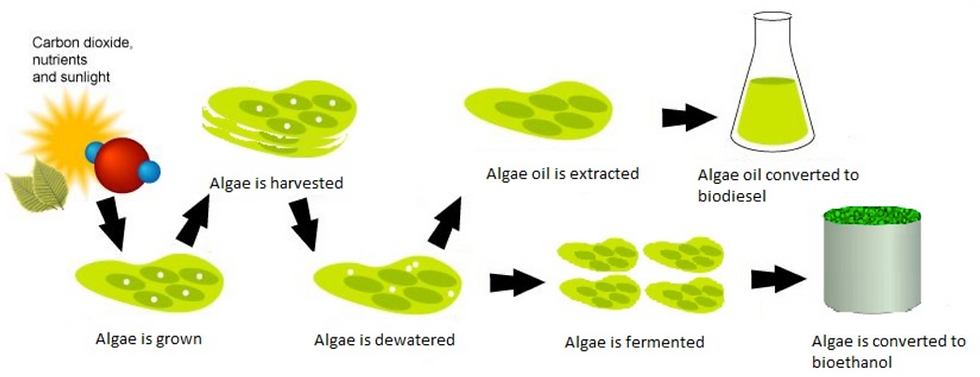
Figure: Bioethanol and Biodiesel production from algal biomass.
Algae can be converted into several types of biofuels via the biochemical and thermochemical routes⁷⁴. A diffused thermochemical approach is gasification, in which algal biomass is typically heated in an oxygen-deprived environment, to generate syngas. Other methods of processing algae are direct combustion, liquefaction (hydrothermal processing) and pyrolysis⁷⁵. An even more attractive option is the production of hydrogen by algae. Classically, hydrogen fuel is produced by processing natural gases with steam, by gasifying coal or by electrically decomposing water into oxygen and hydrogen (electrolysis). These are all energy intensive processes that require high temperatures (>840 °C) and are not environmental friendly⁷⁷. On the other hand, algae can be used to biochemically process water into hydrogen, via several mechanisms (photolysis being the most common).
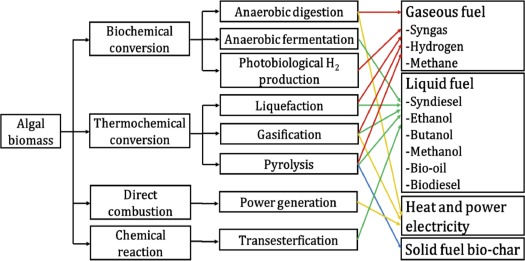
Figure: All the different routes from Algae to biofuel⁷⁴.
Fourth-generation biofuels
In fourth-generation biofuels, genetically modified microorganisms such as microalgae, yeast, fungi and cyanobacteria are utilized as sources. In addition to genetic modification, some fourth-generation technologies involve usual methods of processing biomass such as pyrolysis (in a temperature range between 400 to 600 °C) and gasification⁷².
A slightly different option is the so-called solar-to-fuel pathways. Solar fuel feedstocks (CO₂, H₂O, and solar energy) can be captured onsite or transported to the refinery. Here, solar energy in the form of heat, electricity or photons is used to convert the CO₂ and H₂O into fuels⁷³.

Figure: A diagram summarising the functioning of a solar refinery⁷³.
Summary: advantages of biofuels
By now, you might be wondering whether biofuels tick all the environmental and economic boxes introduced in section 1. As we saw in the previous section, fuel produced from biomass has three main virtues:
Although one of the byproducts of biofuel combustion is CO₂, biomass is commonly considered carbon-neutral, because the amount of carbon released into the atmosphere is the same that had been previously extracted from it by plants⁴⁶. Moreover, biofuels such as hydrogen from algae cultivation very high (if not infinite) H/C ratio. Because hydrogen combusts faster than any other element and produces only water as a reaction product, the higher the H/C ratio, the higher the energy efficiency of the fuel and lower the CO2 emissions from its combustion⁷¹;
In principle, since the biological material input can be grown on human time scales, biomass is a renewable resource that has the potential of being sustainably developed in the future;
The production of biofuels represents an effective way of transforming some forms of waste into valuable energy resources⁴⁷;
For many countries, biofuels might be a one of the few if not the only domestic energy resource⁴⁸;
Biomass price is structurally more stable than petrol, because of the predictability of its supply. This factor might become increasingly more important as fossil fuel prices rise⁴⁹.
In the light of these advantages over conventional fuels, it does not surprise to discover that the European Council in March 2007 recommended that all Member States increase to 10% the share of biofuels in the transport sector by 2020⁵⁰.
However, “all that glisters is not gold” in the world of renewable energy, and even such a promising resource as biomass might disappoint those who are hoping for long-term, environmentally and financially sustainable alternatives to petrol and diesel.
4. Challenges and criticism
Food security: food vs fuel
One of the challenges of using biomass as a fuel source is that biomass is grown on land that could be used for food crops. The limited amount of land and consequently food is even more dramatic if we remind ourselves of the number of chronically hungry people around the world (815 million, i.e. 11% of the world population according to a 2016 FAO’s report⁵²).
Crop inputs for the biofuel industry have included the 12 most common agricultural plants: Barley, cassava, maize, potato, rapeseed, rice, rye, sorghum, soybean, sugar beet, sugar cane, and wheat. Cereals, in particular, are the most common food source for humans and livestock⁵³, and their availability, as well as their prices, are tightly connected to global food security. In some third world countries, there has already been friction between the desire to grow biomass for biofuel production and the necessity of growing food crops⁵⁴. For this reason, some have argued that it is necessary to define the fraction of farmland that could be used for the production of biofuels⁵⁴.
Water footprint
Water is another limiting factor both for human well-being and for bioenergy production, and this — it goes without saying — has generated debates. In fact, as shown in a recent study, the water footprint of 1 litre of biofuel ranges from 1,400 to 20,000 litres of water. Meanwhile, because of climate change, humanity is expected to need water as badly as ever. The importance of water supplies does not impact only the obvious need for drinking water meet the basic human need for water, but also the conservation of rivers, streams, lakes, and aquifers and their respective ecosystems⁵⁵.
Ecosystem impact: are biofuels really green?
Land and water are not the only victims of biomass cultivation for energy. As pointed out in a stark but effective review in 2008⁵⁷:
A key factor affecting biofuel efficacy is whether native ecosystems are destroyed to produce the biofuels. For example, regardless of how effective sugarcane is for producing ethanol, its benefits quickly diminish if carbon-rich tropical forests are being razed to make the sugarcane fields, thereby causing vast greenhouse-gas emission increases⁵⁸. Such comparisons become even more lopsided if the full environmental benefits of tropical forests — for example, for biodiversity conservation, hydrological functioning, and soil protection — are included⁵⁹.
Not to add the impact of the degenerative practices that conventional agriculture (both for food and for biofuel) has had on topsoil, aquifers, ecological functions and micronutrient content in the last century⁶⁰.
Another environmental impact is related to trace-gas emissions. For instance, corn or rapeseed emit nitrous oxide (an important greenhouse gas) due to their nitrogen fertiliser requirements.
Overall, the ecological and environmental impact of 26 types of first-generation biofuels has been compared, and the findings are not promising:
Most (21 out of 26) biofuels reduce greenhouse- gas emissions by more than 30% relative to gasoline. But nearly half (12 out of 26) of the biofuels — including the economically most important ones, namely U.S. corn ethanol, Brazilian sugarcane ethanol and soy diesel, and Malaysian palm-oil diesel — have greater aggregate environmental costs than do fossil fuels.⁵⁹

Figure: Greenhouse-gas emissions are plotted against overall environmental impacts of 29 transport fuels, scaled relative to gasoline. The origin of biofuels produced outside Switzerland is indicated by country codes: Brazil (BR), China (CN), European Union (EU), France (FR), and Malaysia (MY). Fuels in the shaded area are considered advantageous in both their overall environmental impacts and greenhouse-gas emissions.⁶¹
Proposed solutions
Several solutions have been proposed to the “food-land-water-ecosystems vs fuel” predicament, some of which have already been partially implemented.
In order to remove the competition with food crops, some plants such as Karanja, Jatropha, Switchgrass and prairie grasses have been promoted as good sources of biomass. They are not a source of human nourishment, can be grown on waste agricultural land and water, and their fertiliser requirements are relatively low compared to other crops⁵³. These type of argument has led to the rise of second-generation fuels, produced from lignocellulosic biomass (woody material and agricultural waste).
Genetic bioengineering of suitable feedstock such as Switchgrass or Myscanthus has been suggested as a way to compensate for the reduced energy yield of these plants when compared to corn, sugar cane, soybeans or other food crops³⁹. Driven by these considerations, in 2003, Poplar became the first tree to have its entire genome sequenced³². Similarly, interplanting suggestions have been advanced, that could reduce the land required³³.
Unfortunately, some of these alternative crops do not rank well in terms of their water footprint. In 200⁸⁵⁵, scientists found out that the lowest water footprints for electricity generation are those of sugar beet, maize, and sugar cane, typical food crops, while rapeseed and jatropha, typical energy crops, are disadvantageous. Similarly, for ethanol, sugar beet and potato are the most favourable. For biodiesel, soybean and rapeseed have the lowest water footprint, while jatropha has the worse. Land and water requirements are not easy to satisfy for land plants, it seems.
Algal biofuel, the one-hit-wonder of the renewable energy jet-set, is another potential alternative to land-based crops. Algae live in water and do not need to subtract precious land to food production. Moreover, their cultivation can be conducted in low-cost salt-water open pond systems⁶¹, which is obviously in much higher availability than clearwater. Algae are also able to fix large amounts of CO2 (1 kg of micro-algae synthesis takes 1.8 kg of CO2)⁷⁵, they have a very high growth rate (they can double in 24 h)⁷⁴, and can also be a rich source of carbon compounds, which can be utilized in biofuels, health supplements, pharmaceuticals, and cosmetics⁷⁷. However, despite billions of dollars were invested in the extraction of biofuel from algae cultivation which was expected to be commercially available by 2012. By 2019, most of the companies who had taken up the challenge have eventually abandoned it, to veer onto financially safer projects. The biggest challenge that has had to be faced is the extraction of moisture from the algae, leaving a dry powder from which the lipids can be separated. This often requires more energy to power the process than the resulting fuel provides (energy balance issue). Hydrogen production, on the other hand, is still in its infancy³³. However, new developments are underway, and although most capital is slowly branching out onto the use of algae for side-products²¹, there is still considerable hope in genetically modified algae⁷⁸.
Social criticism: are biofuels worth our efforts, after all?
Last, but not least, a severe critique of biofuels comes from environmental activists⁶², as well as the advocates of ecosocialism⁶³, degrowth⁶⁴, and any movements that emphasize the need to reduce energy consumption and therefore energy demand⁶⁵. While biofuels might represent a valid alternative to fossil fuels in the short run, they might end up encouraging a “business-as-usual”⁶⁶ attitude to the climate crisis. Moreover, some observers point out that first world countries are using technological advances in energy production as a way to enforce their economic dominance over poorer nations⁶⁷. This has also been linked to the rise of inequality within society⁶⁸.
One of the most recent scientific reviews⁶⁹ identified four major research challenges in which improvements are needed, if biofuels are to be considered a realistic alternative to fossil fuels:
The processing of agricultural organic matter needs to be made more efficient, by improving the way enzymes are used;
genetically selected and modified microbes should be found and employed to make the fermentation of sugars used in anaerobic methods more productive;
the number of steps involved in all processes should be reduced, soas to reduce production costs;
Recycling, Reduction and Reuse should be the cornerstone of new production, research and distribution strategies — so as to reduce energy demand and minimise environmental impacts.
Are these utopian goals? Is the challenge worth investing precious time and resources in a time of global crisis?
References
The scientific articles and all the other references corresponding to the numbered footnotes can be opened by clicking on the link below. They are in a google doc format. I suggest that you open them in a separate tab (by pressing CTRL+left-click) so that you might refer back to them while you read the article, without the need to scroll back and forth.
コメント