There has been lots of talk, in regenerative agriculture, about syntropic agroforestry. Many of you will have noticed that there is very little (written or video) English material available for those who would like a systematic description of the basic concepts and underlying principles of this method. Throughout the last year, I have been researching syntropic agriculture as an external observer, in order to understand this method - that is inspired by the observation of the indigenous populations of the Amazon, and was formalised and brought to the West by the pioneering work of Ernst Gotsch in Brazil and Portugal.
This post and the ones that follow are an attempt to offer my personal perspective on the topic, with no intention of being authoritative or comprehensive. I haven’t had the pleasure of managing a syntropic system nor meeting Ernst personally yet. The information that I have gathered is the result of several conversations with colleagues and friends who have worked extensively on syntropic systems, as well as watching interviews and reading books, notes and other material available in Portuguese and English.
The syntropic or successional approach to agroforestry outlines a way of interacting with ecosystems which marks a step in the right direction: the ecological (not social/cultural) indigenisation of humans and agriculture. If we put aside cultural factors, what is the biological and ecological role of humans within the ecosystems they are part of? Is it possible to attune what we call agriculture to the processes that humans would engage with in the wild, as part of a complex and dynamic ecological mechanism? As I see it, the uniqueness of Syntropic agriculture lies in this ecological perspective, as opposed to the utilitarian and culturally anthropocentric one which is typical of modern agriculture. That such an approach should strike us as revolutionary gives us a measure of our deep separation not only from our ecosystemic role, but also from the integrated relationship that indigenous cultures have with the land.
1. Ecological succession
Before we can even start to talk about syntropic agroforestry, I believe it is necessary to have a clear understanding of one of the most fascinating and totally spontaneous processes that take place on our planet: ecological succession.
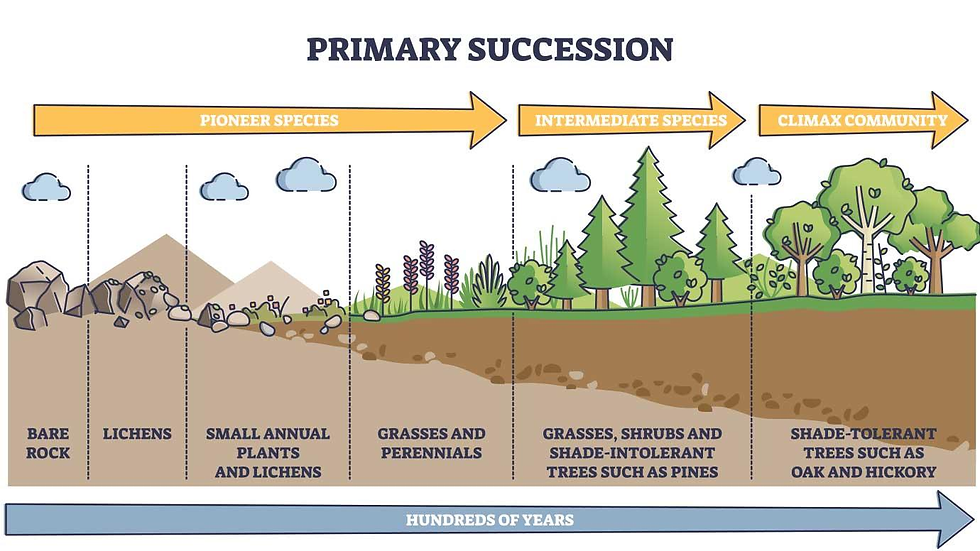
Colonisation
In the wild, a degraded soil gets quickly colonised by a diverse series of plants, animals and microorganisms. Initially, microbes are the only life forms, and they start to make the minerals contained in rocks soluble; they also increase the availability of oxygen and CO2, thus preparing the ground for plants. The first plants are herbaceous (i.e., without woody tissues), these include ephemeral, annuals, biennials and finally perennials. They are able to adapt to extreme conditions, such as low fertility and water availability. As pioneers, these plants fulfil their role by accumulating minerals from deep down the soil profile; they partner with bacteria including nitrogen-fixing ones; they add organic matter to the soil both by root exudates and with their tissue when they die for the winter or at the end of their life cycle. This phase can be named “colonisation”, and (in syntropic jargon) it represents the “placenta” of the ecosystem.
Accumulation
With their work, herbaceous plants prepare the soil for the arrival of more complex species, such as pioneer shrubs and trees. These usually arrive into the system via the wind. Shrubs and trees carry on accumulating and cycling minerals and organic matter on the surface of the soil, adding in the lignin contained in their tissues - a compound that decomposes a lot more slowly than cellulose and induces *humification* (the creation of humus, or stable organic matter). The resulting organic material creates an ideal food and habitat for fungi; these, in turn, respond by intensifying their mycorrhizal network through which plants communicate and trade nutrients.
Shrubs and trees are also more effective at improving soil, because of their deeper roots and their ability to provide shade during the hotter months, thus creating less extreme conditions for plants in the undergrowth. As a result, herbaceous pioneers start to be less abundant and are replaced by more demanding plants, more similar to our common vegetables and thus rich in carbohydrates and proteins.
This is the accumulation phase. What is being accumulated is natural capital, measured in terms of biodiversity, the complexity that characterises the relationships among the living organisms present in the system, and the forms in which energy is being stored (fertility, organic matter). Such an accumulation corresponds to an increasing level of organisation, which can be technically defined as syntropy (or negentropy) - the inverse of entropy, the quantity that measures the level of disorder characteristic of non-living systems.
The accumulation phase marks the arrival of the first fruiting plants; these represent a source of complex sugars that enriches the food dynamics within the ecosystem, and also attract larger animals into the system, birds and mammals in particular. Medium and large size animals (wild bore, deer, etc.) fertilise the soil with their droppings and create ecological niches with their disturbance action, thus allowing the system to further increase in complexity. Mammals and birds also bring the seeds of more demanding plants, such as fruit and nut trees, which indicate the approach to the abundance phase.
Abundance
The abundance or climax phase is characterised by plants which populate all the layers or strata of three-dimensional space (low, medium, high, emergent), as well as having a diverse range of life-cycles (ephemeral, annual, perennial). Above all, we see an increase in diversity, complexity and the accumulation of energy in the form of organic compounds: humus, protein, carbohydrates, sugars, fats, etc - both above and below ground. Typical of this phase are fruit, nut and timber trees, productive climbers, starch-rich roots and a diverse fauna including mammals, reptiles, birds, insects that feed on vegetables and predate on one another.
Thus, the stability of the ecosystem has increased alongside three main factors: biodiversity (of microbes, plants, animals); complexity in the interaction between all the elements (trees communicating via mycorrhizal networks, food chains above and below ground, etc.); and finally soil fertility. The latter is the common thread throughout the entire ecological succession, because it is in the soil that all the efforts of the initial phases focus; and it is in the soil that minerals, organic matter, water and the main core of biodiversity are developed over time.
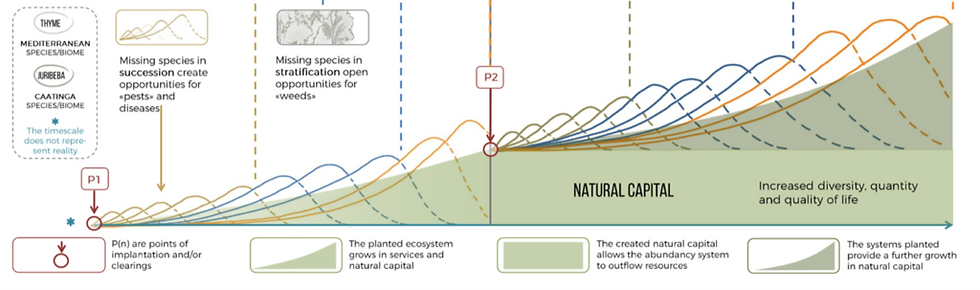
Dynamical equilibrium and patch dynamics
This is clearly a simplified view of one of the most important and defining processes that take place on earth. In fact, each phase has within itself a level of colonisation, accumulation and climax. Some species act as turning points within this internal dynamics. Brambles (Rubus fruticosus) are such an example. These establish themselves as a pioneer shrub, towards the end of the colonising phase; they spread fast by letting the tip of their arching branches root into the soil. With their flowers, brambles attract pollinating insects, and with their fruits they entice birds that introduce the seeds of more demanding species with their droppings. The shrub and trees thus imported germinate among the brambles, protected from herbivores by their vicious thorns. Thanks to this characteristic behaviour, brambles colonise gaps within woodland and field edges, thus marking a turning point between herbaceous and tree species, as well as between tough and delicate ones.
This process can take place also in a mature ecosystem, whenever a gap is created by the disturbance caused by an animal or a geological or climatic event. In such gaps the soil gets disturbed and the level of light is typical of an earlier ecological phase compared to the surrounding areas. When this happens, the neighbouring patches and the existing soil act as an inoculant of biodiversity and fertility, thus stimulating a repopulation of the gap which is faster and adds additional complexity to the entire ecosystem. This process (called secondary succession) repeats itself at random intervals, thus creating a patch dynamics that makes the ecosystem resilient and characterised by a dynamical equilibrium, maintained by an alternation of disturbance and consequent accumulation of natural capital.
2. The growth “pulse”
A mechanism, in particular, is worth highlighting within this process.
Plants respond to pruning or herbivory by reallocating carbohydrates to the below-ground parts of the plant and increasing exudation rates. This, in turn, increases microbial activity in the rhizosphere and mineralisation (more microbes make more soluble nutrients available). Where mycorrhizal networks are well established, a biochemical signal (which some believe is mediated by the plant hormone gibberellic acid) is also sent to the neighbouring plants.
This combined microbiological and biochemical/hormonal stimulation has a triggering effect on the surrounding vegetation, which experiences more nutrient availability, and is stimulated to grow more vigorously, photosynthesise more and set more flowers and fruits.
Thus, when the canopy of a plant is pruned, not only light is introduced to the lower layers, but a “growth pulse” is propagated into the system, and felt by the neighbouring plants.
The plant that is pruned, harvested or mechanically damaged is also rejuvenated, and can live a lot longer than it would if it weren’t pruned. This process is hormonally reversed when plants develop flowers and start to ripen their seeds. At this stage, they become more demanding (for water and nutrients) and competitive.
When the first westerners colonised North America, they reported that after clearing a forested area, for a few years they were blessed with the best soil and growing conditions they had and would ever experience. What they were doing was creating a gap in a well-established ecosystem. In the gap, soil is at its peak fertility, the extra light creates the perfect conditions for vegetable and fruit production, and the pulse generated by the felling and the pruning enhances this effect. If one were to plant young trees alongside vegetables in such a gap (like indigenous Amazonian tribes used to do) this would gradually recreate forest conditions in that same patch. Then a new patch can be cleared, thus effectively growing light-, water- and fertility-demanding crops within a forest, without affecting the stability of the latter. This eye-opening realisation lays the basis for a way of growing vegetables that is in harmony with natural processes.
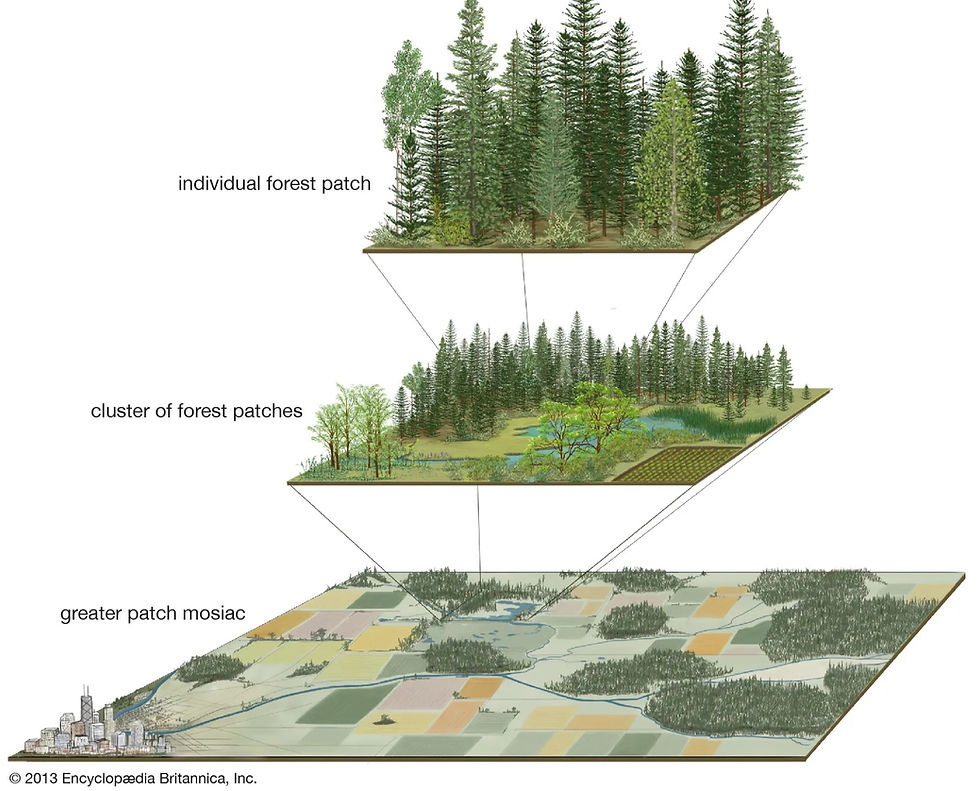
3. The principles of Syntropic agriculture
Syntropic agriculture is rooted in the careful observation of the ecological and physiological phenomena described above in various geoclimatic contexts. The syntropic approach is focussed on preserving these mechanisms and take advantage of their spontaneous character to trigger and accelerate the process that leads from a degraded, disturbed or ordinary soil to an abundant ecosystem.
The theoretical framework of syntropic agriculture is founded on a series of principles that codify natural, ecological processes and make them utilisable in productive contexts.
It is indeed possible to mimic natural ecosystems closely. If we observe the successional dynamics discussed in the above, we notice that a living system develops its complexity, diversity and fertility over four dimensions: space and time. Energy is stored in the form of organic matter and resilience is built upon complexity and functional redundancy. Throughout the ecological succession process, an ecosystem increases its level of organisation, encoded in the physical quantity called syntropy. The increase in syntropy is a lot less common in the Universe than one might realise, and only living systems show a marked tendency to do so, while inorganic ones always tend to a constant increase in (internal) entropy.
A formal theory of syntropy was proposed by one of the most talented mathematicians of his era - Luigi Fantappiè. In the 1940s, he started working on a unified theory of biology and physics, which would have Einstein’s relativity as a limiting case and the origin of life as natural emergent phenomenon. His theory was based on the interaction of syntropy and entropy, and the existence of three levels of time. I won’t go into this (very complex and controversial) theory, which costed Fantappiè his reputation, but I will leave it to the interested reader to delve deeper into his fascinating elucubrations.
In practice, syntropic practitioners have developed a series of techniques that reproduce what would happen in the wild, but using design and observation to accelerate the ecological processes and make the resulting system highly productive.
In particular, by studying ecological succession, it is possible to become increasingly independent on external inputs: after all, the succession process described above only needs a few microbes, a mineral soil, water and solar energy to trigger a series of transformations that leads to mature forest. In syntropic approaches, then, it is pointed out how external inputs, if necessary, can be kept to a bare minimum, because the plants themselves are used to create fertility via photosynthesis and their microbial partnerships. The regeneration of agricultural ecosystems involves the accumulation of fertility in the form of biomass (which gets ideally converted into stable soil organic matter), and microbiological diversity and activity. This accumulation can be delegated to pioneer plants which get chosen, planted and pruned strategically, to mimic what would happen in the wild and dramatically accelerate the process of ecological succession and enhance the resulting abundance. Such a process would take place anyway, but on much slower time scales compared to what we aim to achieve in agricultural systems.
By engaging in this strategy, humans reclaim their role as large mammals, whose function is mobilising and cycling organic matter by interacting with a multitude of trophic levels within the ecosystem. This has two consequences. Firstly, the humble recognition that we are not the only intelligent component of a mechanical system, but we are part of a system that is itself intelligent. Secondly, the definition of principles and the development of techniques that leverage the spontaneous character of ecological processes.
The key principles of Syntropic agriculture are:
Mimicking the natural ecosystem of the site in structure, dynamic and function
Avoiding external inputs other than knowledge and planting material (seeds or plants)
Relying on plant’s ability to mobilise and recycle their own nutrients and water according to their needs
Designing and managing a system so that its natural capital (quantity and quality of consolidated life) increases and contributes to increasing the syntropy of the macro-organism Planet Earth.
These principles can be applied through a series of guidelines and techniques that can be grouped in four groups:
Four-dimensional stratification in time and space
Planting and selective/strategic pruning of pioneer plants that fulfil a service role (humification, shading, accumulation and cycling of water, biomass creation, habitat for insects and microbes)
Application of the principles of regenerative agriculture: avoiding synthetic chemicals and soil disturbance, keeping the soil covered, encouraging biodiversity and maximising photosynthesis
Concentration of energy and biomass production
So, how does a syntropic system work and how to establish one from scratch? How do we apply syntropic methods to commercial operations? These are questions for another post. Stay tuned!
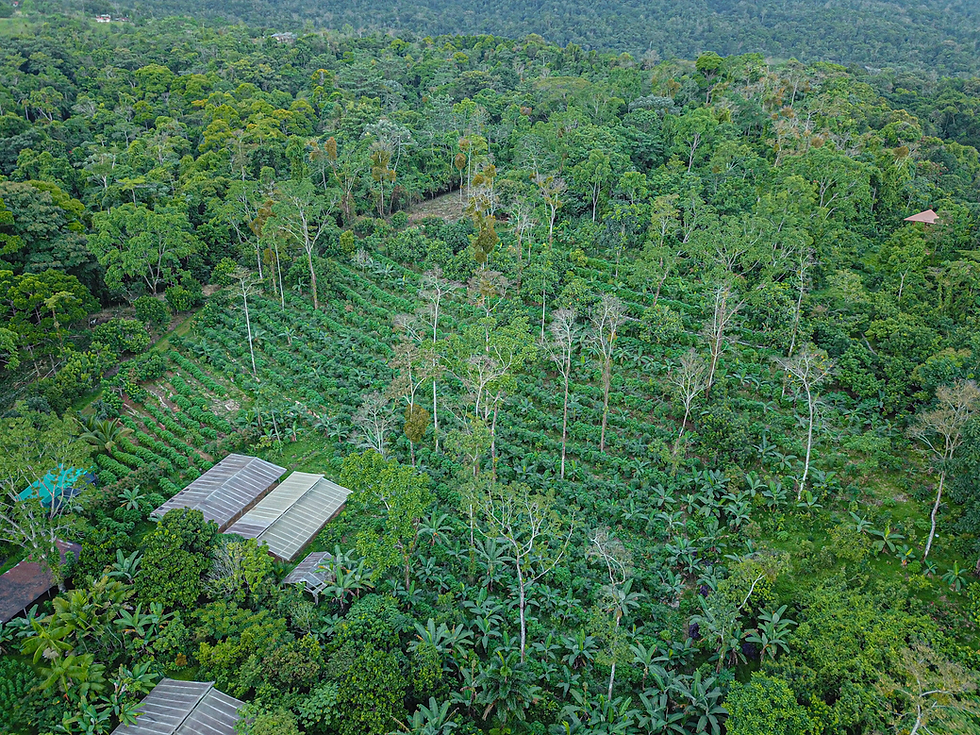
Photo Credit: Finca Luna Nueva, Scott Gallant
コメント